What are superconductors?
1992/05/01 Otaolaurretxi, Jon Iturria: Elhuyar aldizkaria
Discovery of the phenomenon
Superconductivity is a phenomenon discovered in 1911 by the Dutch physicist Heike Kamerlingh Onnes. By cooling a sample of mercury to 4 degrees Kelvin (-273,16ºC is the temperature of 0 K) he realized that the electrical resistance of the material disappeared sharply. In other words, mercury is like any other conventional electric conductor at temperatures above 4 K, but when it drops below the “critical temperature” of 4 K, it does not put any obstacle to the passage of the electric current, it is said to be a superrconductor.
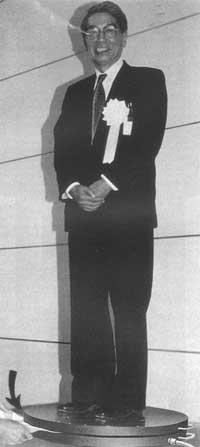
The same phenomenon occurred with other metals. For example, lead has a critical temperature of 7.2 K, 3.7 K tin, 9.2 niobium, etc. Since the 1950s, metal alloys have also reached superconductivity: For example in alloy Nb3Sn (18 K) and alloy Nb 3 Ge (23 K).
However, keep in mind that at low temperatures not all materials are superconductors. For example, copper, being a good conductor at room temperature, although at very low temperatures it is not a superconductor and the same happens with almost all insulators and semiconductors.
Superconductivity in recent times
After discovering the phenomenon at the beginning of the century, physicists presented their first theory in 1957. The first application was reached in the 1960s, in superconductor magnets that produced high-intensity magnetic fields (currently used in many advanced technology appliances, magnetic resonance machines, particle accelerators, etc. ).
In 1986 the need for very low temperatures was abandoned. Although until then the highest critical temperature was 23K, the rise of the limit to 125K has changed a lot. Therefore, from 1986 in laboratories this type of research fever has occupied people working in superconductivity, system of accumulation of electricity, magnetic levitation train, computer of superconductors, etc. by getting. Over 18,000 scientists have published in the last six years on this subject.
Superconductivity Theory
In 1957 the Americans John Bardeen, Leon Cooper and John Schrieffer published a theory about the whole phenomenon, called “BCS”.
To show the content of the theory and understand what happens to the superrconductor, so that we say something about the environment. Like any other matter, the solid is formed by atoms and the atom has nucleus rotating around the electrons.
The core of a copper atom, for example, has 29 protons (or positive electric charge) and 34 or 36 neutrons, depending on the type of isotope. In an isolated copper atom, 29 electrons (or negative electric charge) are rotating around the core in layers. The atom in general is electrically neutral in itself, as it has as many protons as electrons.
However, in a common copper sample, atoms have peculiarities. On the one hand they are regularly aligned with each other forming a crystalline net, and on the other hand each atom is ionized, that is, one of its electrons is delocated in the net. In addition, these loose electrons can circulate freely within a tube like fluids. Therefore, if this material is connected to the terminals of the battery or alternator, this set of electrons begins to work forming electrical current.
However, if the passage of electrons in the common conductor is accurately observed, it is observed that each electron has an individual behavior. During its journey it collides and rubs with the ions of the crystalline network. It thus loses some of its energy and emits heat. On a macroscopic scale it is said that the material counterposes the resistance to the passage of the current.
In the superconductor this resistance disappears because electrons lose their individual behavior. Each attracts the positive ions of the network when moving and these in turn attract another electron. This link between the two electrons generates pairs of electrons that cannot break at very low temperatures. The two electrons of the pair go at once, although the distance between the two is relatively large (thousands of angström; angströma = 10 -10 m). In a place of superconducting material, in addition, there are millions of pairs of electrons.
The result is surprising. In the superconductor the electric current is not formed by electrons, but by pairs of electrons. This is the fundamental difference, as the quantum behaviors of these objects are different. Couples can be grouped into the same group situation forming a kind of superconducting fluid in which there are no shocks or obstacles to individual electrons.
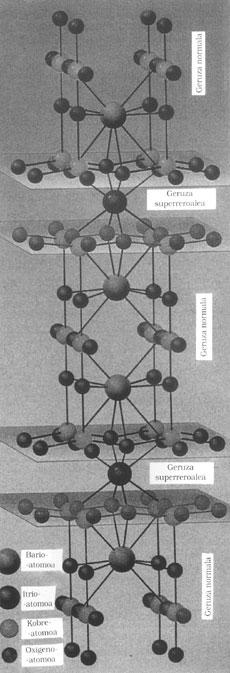
Bardeen, Cooper and Schrieffer have given this simple two-stage image in the mathematical language of solids physics. In a first stage, in interaction with the crystalline network, pairs of electrons would be formed and in the second stage they would condense to the same group state.
Evolution of critical temperature
A major obstacle for superconductors is the need to be at very cold temperatures in order to function. Until 1986, temperatures of up to 23K (-250ºC) were needed to detect
the phenomenon of superconductivity, which required costly liquid helium cooling systems.
In 1986 and 1987, researchers Alex Muller and Georg Bednorz have detected superconductivity at much higher critical temperatures in IBM laboratories in Zurich. 90 K and 125 K raised the critical temperature mark with copper oxides. Thanks to this, today you can work within a more economical liquid nitrogen technology, but it has also had other effects. Researchers are flying crazy to achieve superconductivity at room temperature. What is the current situation?
To answer this question it is necessary to clarify four points. First, we need to clarify how superconductive materials are obtained; second, what we know about their structure; third, how superconductivity is explained at high temperature and finally, what applications consist of.
Obtaining materials
Superconductive materials can be obtained fairly easily by synthesis. To obtain YB 2 Cu 3 O 7 (barium oxide, yttrium and copper), for example, the components must be dosed in the proportion indicated in the formula. The materials are molded and the powder is heated to about 1000 ° C. Small glass beads are obtained that are roasted hot and stuck together.
However, in the process of obtaining these materials many factors intervene and it has been possible that the compound of the same formula (Tl 2 Ba 2 CuO 6) is not superconducting for some and has a critical temperature of 90 K for others. It was later observed that the synthesis conditions are of vital importance in the characteristics of the compounds.
Stratified structure
The compounds found since 1986 present a stratigraphic structure with numerous defects. Consider the example of the above-mentioned compound YB 2 Cu 3 O 7. The key to this structure is the layer formed by two CuO 2 planes, in which each copper atom is combined with two oxygen atoms by chemical bonds. These two planes of CuO 2 are separated by atoms of yttrium. The whole, for its part, constitutes the layer that constitutes the headquarters of superconductivity. Between two superconductive layers is interspersed a normal layer with CuO x chains (between x 0 and 1), barium and oxygen atoms. In the whole, therefore, the superconductive and conventional layers are interspersed.
The role of the common layers is not yet decided. Some consider it a load tank for supplying superconducting layers. Others believe, however, that because of its closeness, this layer also becomes superrconductor. However, the problem is not yet solved.
Moreover, errors in these compounds are very debatable. They can be specific errors (for example, that a hydrogen atom is insufficient or excessive at a specific point in the network), but they can also be extended (an error in the rotation of layers or a set of atoms not distributed homogeneously). It has been proven that the role of oxygen shortage is very important, since the absence of either atom reinforces superconductivity. It is unclear to what extent layers and structural defects influence the phenomenon.
Superconductivity mechanism
When asked about this issue, physicists first refer to disorder. Then there is talk of abundance to explain superconductivity at high temperature. In reality, physicists do not know the mechanism of superconductivity at high critical temperature. And they have invented many models to express the phenomenon.
In the tests carried out since 1987, strange phenomena have been detected in pairs of electrons carrying electric current, such as small distances between the electrons of the pair (dozens of angström, while in the classic model BCS are one hundred thousand times greater). J.V. Thanks to the tests carried out by Uemura at Columbia University (New York), the “depth of the magnetic field” has been measured, that is, to what extent the magnetic field has entered a superreroal sample. It has been measured in families of high-temperature critical superconductors, but also in other exotic materials (organic superconductors, uranium compounds, etc.) found in the last twenty years.
Uemura has been able to verify that exotic hus superconductors at high critical temperature have the characteristic that their critical temperature is inversely proportional to the square of the depth of the magnetic field. This suggests to physicists the existence of an electron pairing mechanism, in which the crystalline net would deform and therefore the electrons of the pair would be very close. Confirming this interpretation, superconductor families would come together and it would be possible to reach higher critical temperatures.
Superconductor Applications
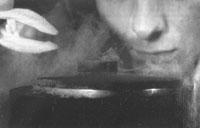
To understand the influence of superconductivity we must think of a world without electrical resistances. The first application that is created is an electric current without resistance. Heat would not be lost in the superconductor thread. In theory, endless movement would be possible and the superreroal current can be maintained for years without losing energy. Therefore, there would be accumulation of electricity.
Another field of use is that of electromagnets. Superconductor coils would allow intense current intensities to circulate generating huge magnetic fields. There are currently limitations to heat generation in electromagnets in conventional conductors.
Barriers to applications
However, superconductivity finds its obstacles in practical applications. Critical temperature is not the only limit. In 1911 Kamerlingh Ones made a lead coil to create large magnetic fields and cooled it with liquid helium up to 4K. Lead has a critical temperature of 7.2 K, but Ones found that if the magnetic field was higher than 0.05 teslas, lead became a normal conductor. The superconductivity is due to the fact that in the materials it appears when the temperature, the magnetic field and the electric current (three parameters therefore to be monitored) are inferior to a certain measure. Each superconducting material has its temperatures, magnetic fields and critical currents and its compliance greatly limits applications.
The last superconductors found are ceramic, that is, formed by a glass called “gasket”. This structure means that the current must pass between the grains and cannot have much intensity. In YBaCuO superconductors, the current density in 1987 was only hundreds of 2 cm amps. From this moment on, the intergranular joints have been improved with respect to superconductivity, reaching densities of 40,000 A/cm 2, without magnetic fields.
Bismuth superconductors seem to have a better future. The material Bi 2 Sr 2 Ca 2 Cu 3 O 8, for example, has a critical temperature of 110 K. The Japanese firm Sumitomo Electric has reached densities of 4 K of 10 5 A/cm 2, but in magnetic field of 25 teslas.
However, last year the Japanese research institute ISTEC has broken a new record. Using 250 YBaCuO tablets, the magnetic fields created by superconductors have managed to lift an inch of the earth with the president of a platform. The CRTBT research center located in Grenoble (France) has reached densities of 8,000 A/cm 2 to 77K and area of 6 teslas.
With these characteristics, detectors and sensors can be prepared. SQUID (Superconducting Quantum Interference Device) can measure very small magnetic fields. Last year, for example, Berkeley Californians presented a SQUID that measured the magnetic field created by the human heart.
However, the future of superconductors is still not entirely clear, but there are applications such as magnetic levitation that will surprise us.
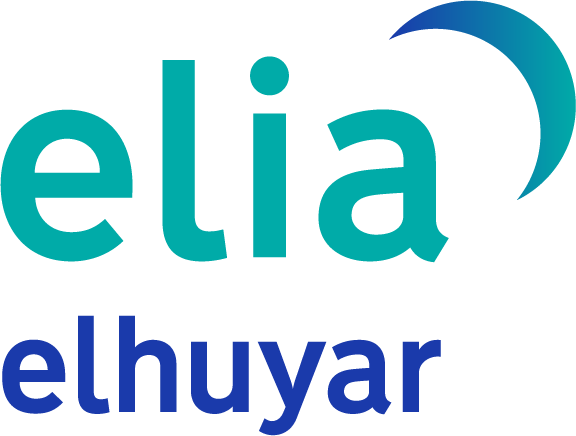
Gai honi buruzko eduki gehiago
Elhuyarrek garatutako teknologia