Beware of Quantum
2024/10/23 Babaze Aizpurua, Antton - Materialen Fisika Zentroko ikertzailea Iturria: Elhuyar aldizkaria
Imagine the gold rings, the neck and the earrings that the grandmother wore on the bedroom shooter, with that characteristic shiny golden color. Anybody would say it's the natural color of gold that we imagine, isn't it? Well, if we threw these grandmother's jewelry and cut it down to nanometric size (making a thousand times smaller than the thickness of a hair), they would no longer send the shiny golden, but red or green! What’s more, depending on the shape of the jewel, they would send one or the other color! [1] What causes this fascinating transformation? This color change is due to a unique property of metallic nanoparticles: plasmo-2 resonance, i.e., electron oscillation when illuminating a metallic nanoparticle (see Figure 1).
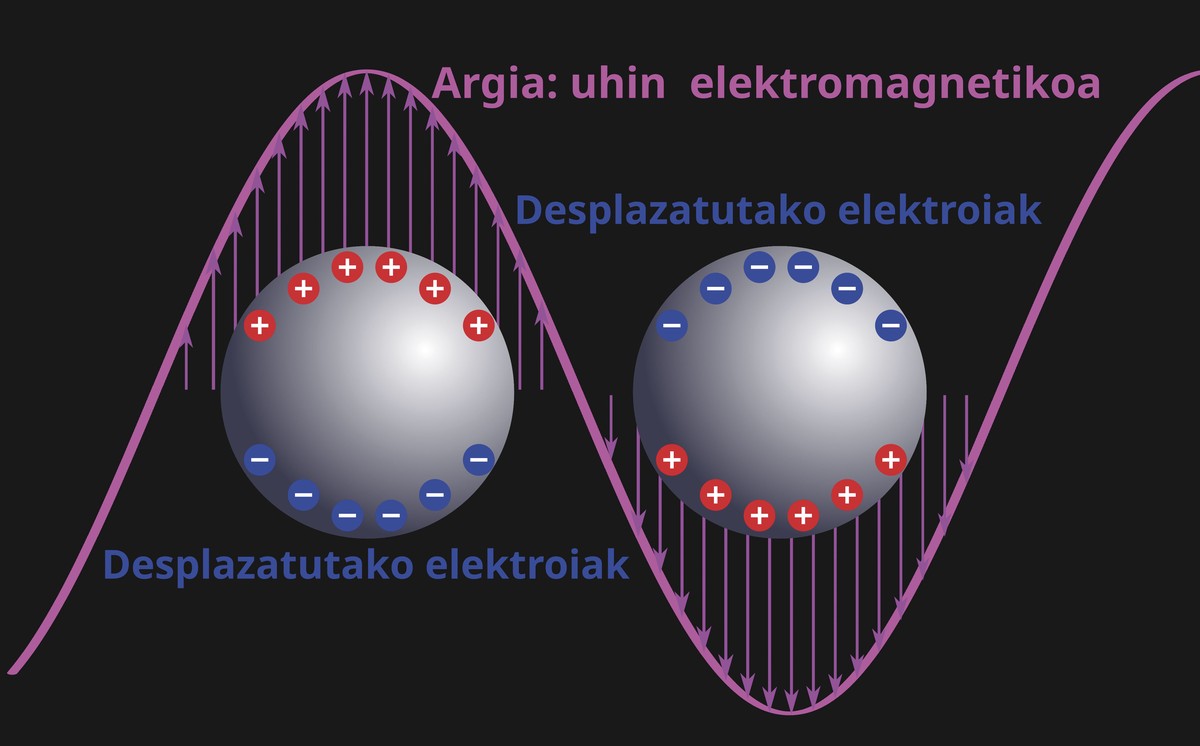
Light is an electromagnetic wave, just like gamma rays or radio waves. The main characteristic of light is the frequency, the number of oscillations that are performed by electromagnetic waves in each second. Frequency plays a fundamental role in the perception of the world, as it is she who determines what we understand by color. Every illuminated body absorbs part of the electromagnetic waves and reflects another part. The eye receives these reflected waves, and through some of the cells that we have in the retina, electrical signals are sent to the brain. The brain then interprets every frequency received as a color. But let's go on with placist resonances.
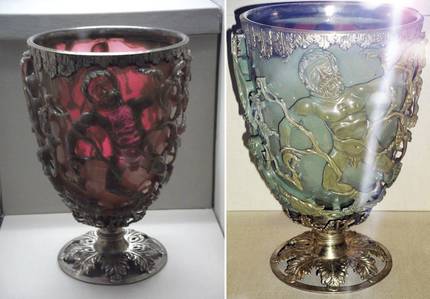
When light affects a metal nanoparticle, the electrons in it oscillate with the frequency of light, like sea waves, and absorb and scatter light to create surprising colors. The oscillations of these electrons are plasmons. However, this phenomenon is not only a scientific curiosity, but has profound implications in different areas of nanotechnology. However, even if it seems strange, the first signs of placistic resonances can be found for more than a millennium. A notable example is the 4th century Licurgo Cup [3], a Roman glass cup that looks green when illuminated ahead and red behind (see figure 2). This trick to change color is due to gold and silver nanoparticles inserted into glass. At that time, metallic nanoparticles are likely to have been inadvertently introduced, but we are now taking advantage of them for modern applications. An ancient relic playing with nanotechnology. Who would say it!
Nanoantennas: light in small spaces!
A fascinating aspect of plasma resonances is the ability to capture light in very small areas, so metal nanoparticles can operate as nanoantenna [4]. Think about the radio antennas that take electromagnetic signals and concentrate them on a receiver; plasmosis does something similar, but light, and they confine it to nanometric sizes. This confinement allows light to be manipulated on a scale much lower than the wavelength, which is fundamental for advanced optical and photonics applications.
But nanoplasmic isn't just transmitting pretty colors. It has far-reaching and revolutionary applications. From improving the efficiency of solar cells to creating very sensitive sensors or improving data storage, placist resonances are at the heart of many technological advances. One of the most anticipated applications of nanoplasmic is cancer treatment [5]. Plasma thermotherapy takes advantage of the heat generated by plasmoplastic resonance. Gold nanoparticles can be channeled to cancer cells, and when they are exposed to the action of light, harmful cells become warm and destroyed without damaging the surrounding healthy tissue. This type of therapy offers less invasive and effective treatment against traditional methods.
Even more interesting next to the molecules!
The properties of plasmosis become even more interesting when they interact with the molecules installed in the vicinity. For example, they play a fundamental role in improving molecular spectroscopies, as placist resonances can amplify the signals emitted by molecules, and so scientists can detect individual molecules [6]. This capacity is useful, for example, for chemical analysis.
In my doctoral dissertation [7], I've researched the interaction between molecules and metallic nanoparticles, analyzing the shocking quantum phenomena that occur when classical physics breaks the law and the quantum world takes over.
Electronic bridges
The first surprising discovery was when I saw that a molecule was placed between two metal nanoparticles [8]. To do this, I didn't use a lab, but long, heavy computer simulations, longer, heavier and fun, I would say. I put the molecule very close to two nanoparticles, at an average of about nanometers, and I sent light to the whole system. I observed that the electrons of one nanoparticle absorbed the energy of light and, passing through the molecule, jumped to the other nanoparticle (see Figure 3). There was no direct contact between the molecule and nanoparticles, but despite that, electrons could move between nanoparticles. It's like electrons teleport from one place to another without physical contact!
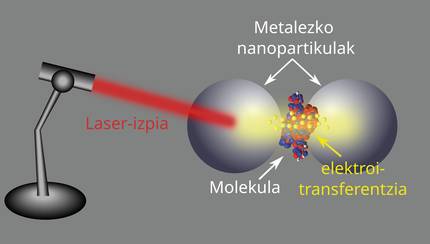
Here comes the magic of quantum mechanics. In fact, electrons are transferred through the tunnel effect. The tunneling effect of electrons is a quantum phenomenon in which electrons can cross a kind of barrier that they cannot classically cross. It is said that they cannot cross it in a classical way, as electrons lack the energy needed to overcome the potential between vacuum and metal. This effect is unparalleled in the macroscopic world in which we are accustomed and can result in a nanometric scale conductance pathway, facilitating access to new electronic devices that are very small but very efficient. Imagine a kind of "invisible bridge," it's just quantum magic!
Emission of prohibited light
Another important finding was that when a molecule is placed next to a metal nanoparticle, the color of the light emitted by the whole system can vary completely. First of all, I lit only nanoparticles, no molecules. Of course, again using computer simulations. I saw that the nanoparticle emitted the color of the light that I was sending to it, that is, using red light, the nanoparticle emitted the color red, and using blue light, the nanoparticle emitted the color blue.
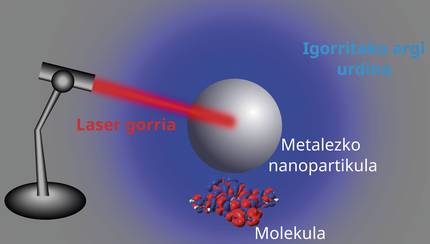
But when I put a molecule next to the nanoparticle, it was a really interesting thing. When I sent the red light, the system could emit blue light. This phenomenon is the result of another quantum effect in which the system absorbs two red (low frequency) photons at the same time and emits a single (most frequently) blue photon. How many things nanoparticles and molecules can do together, no!
The influence of nanoplasmmónica is evident and important today. Who would have believed that such a negligible thing could have such a significant effect? The next time you see a simple piece of metal, remember that beneath your surface there is a world of infinite colors and possibilities. The findings announced in my doctoral dissertation coincide with this fascinating, ever-evolving puzzle, but will they help advance nanometric electronic devices? Only time will tell.
Bibliography
[1] N. J. Halas, S. Lal, W.S Chang, S. Link, and P. Nordlander (2011) “Plasmons in strongly coupled metallic nanostructures”. Chemical reviews, 111(6), 3913-3961.
[2] L. Novotny and B. Hecht (2012)Principles of nano-optics. Cambridge University Press.
[3] I. Freestone N. Mr. Sax and C. Higgitt (2007). “The Lycurgus cup—a roman nanotechnology.” Gold bulletin 40 270-277.
[4] L. Novotny and N. Van Hulst (2011) “Antennas for light”. Nature photonics, 5(2), 83-90.
[5] R. Bardhan, S. Lal A. Joshi and N. J. Halas (2011) “Theranostic nanoshells: from probe design to imaging and treatment of cancer”. Accounts of chemical research, 44(10), 936-946.
[6] F. Benz, M. K. Schmidt A. Dreismann, R. Chikkaraddy, Y. Zhang, A. Demetriadou, ... and J. J. Baumberg (2016) “Single-molecule optomechanics in picocavities”. Science, 354(6313), 726729.
[7] A. Protection (2022). “Quantum Many-Body Effects in the Optoelectronic Response of Plasmonic Nanostructures and their Coupling to Quantum Emitters”. Doctoral dissertation. University of the Basque Country.
[8] A. Babaze R. Stephen, A Doctor G. Borisov and J. Aizpurua (2021) “Electronic exciton–plasmon coupling in a nanocavity beyond the electromagnetic interaction picture”. Nano Letters, 21(19), 8466-8473.
[9] A. Babaze R. Stephen, J. Aizpurua and A. Dr. G. Borisov 2020. “Reman-harmonic generation from a quantum emitter coupled to metallic nanoantenna”. ACS photonics, 7(3), 701-713.
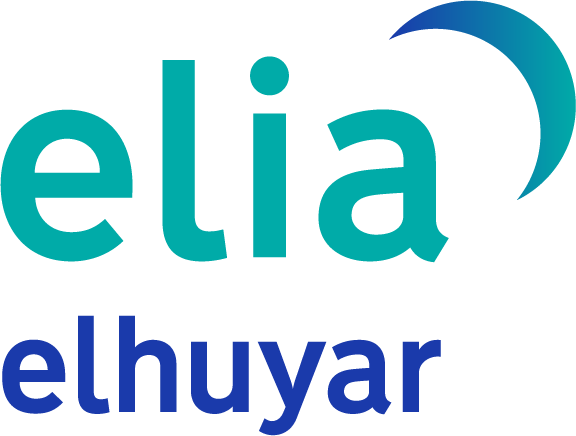
Gai honi buruzko eduki gehiago
Elhuyarrek garatutako teknologia