CAF-Elhuyar 2018: Symphony of black holes
2018/05/16 Miguel Querejeta Pérez Iturria: Elhuyar aldizkaria
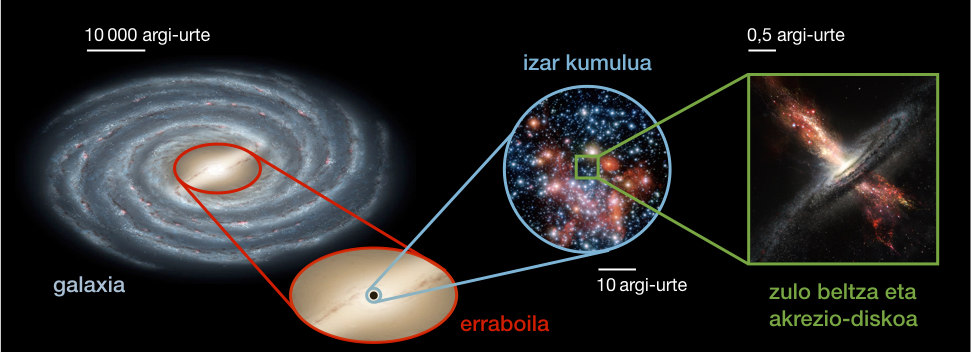
Most galaxies have a giant black hole in the center. And those huge black holes, which hide inside the mass of millions of stars and light cannot escape them either. But these giant black holes have a great influence on galaxies, as their large gravitational fields attract the matter around them and can cause strong changes in hotel galaxies. The aim of this doctoral thesis was to analyze the interaction between giant black holes and galaxies. Welcome to this curious cosmic spectacle: the symphony of black holes begins.
The black heart of galaxies
We could say that all that is around us is the dust of the stars and most of the atoms that form our body. To create heavy elements it is necessary that cosmic explosions occur as explosions of massive stars such as supernovae or collisions of neutron stars. These phenomena emit so much energy, in which elementary particles can be reorganized into heavy elements, while the explosion scatters through the galaxy, resulting in new stars and planets. In addition, the explosions of massive stars leave behind a black hole.
But if black holes also catch the light, how is it possible to find and analyze black holes? We cannot see what happens inside the hole, but we can measure the influence of black holes on them, that is, we analyze black holes indirectly by investigating their consequences. Over the past decade, carefully following the movement of the stars in the center of our Milky Way, it was possible to deduce the existence of a huge black hole. Newton's laws tell us that stars that are orbiting around a point will spin faster when the mass of the center is greater, and that's what they concluded: the equivalent of billions of stars is concentrated at the core of the Milky Way (Figure 1). What is at the center of our galaxy is the giant black hole closest to us, but as we are immersed in our galaxy, it is not easy to sense the global consequences of the black hole. When we are in the forest, we can see the trees with great precision, but at the same time lose the perspective of the whole forest. Therefore, in this doctoral thesis we have observed a galaxy outside the Milky Way, the famous spiral M51.
Galactic Journey to the Black Hole
The organization of gas is of great importance, as it conditions the creation of new stars. And in the M51 galaxy the gas is not still, but travels towards the center, which is the first great result of this doctoral thesis. To conclude this it is essential to know well the gravitational field of the galaxy. This area of gravitation can be studied thanks to images from the NASA Spitzer Space Telescope, which receives infrared waves and reflect the position of the mass of the stars. It is easy to calculate the gravitational field generated by this mass and the effect it will have on the gas. But this would also require a precise gas map, the most accurate map to date.
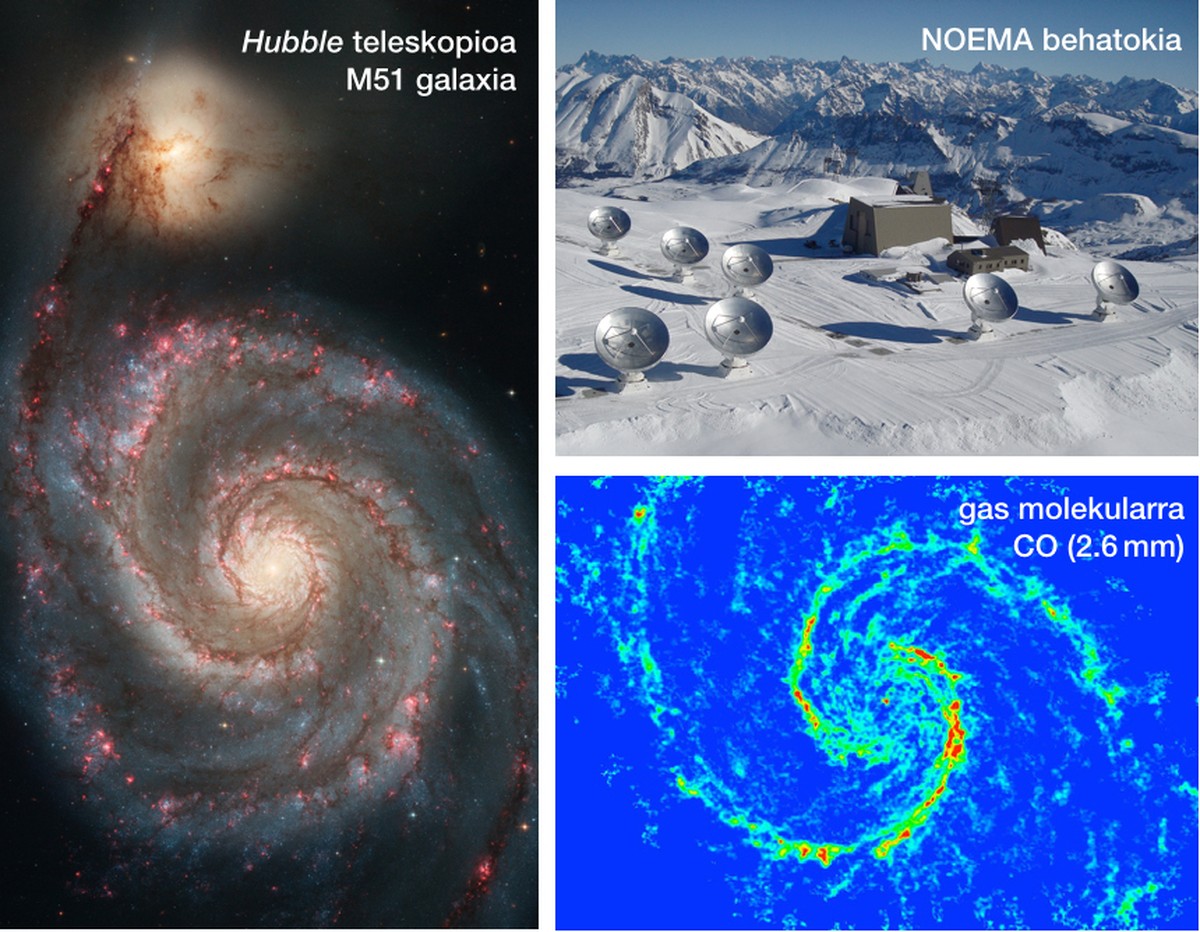
In the French Alps, at 2,550 meters high, the parabolic antennas of the NOEMA interferometer observe the universe. In winter, the hills around the antennas are full of skiers, as astronomers use telescopes to climb these telescopes. Parabolic antennas receive radio waves to study the situation and movement of molecular gas. In addition, by combining the information collected by different antennas, we can obtain very high resolution images. Molecules emit energy at certain wavelengths as a result of quantum transitions; the fundamental transition of the CO molecule, for example, has a wavelength of 2.6 millimeters. In the M51 galaxy we have detected this emission line using the NOEMA, with the highest precision achieved so far in an external galaxy (Figure 2).
Therefore, using as a tracer the line corresponding to the 2.6 mm of the CO molecule, we have been able to measure the movement of the gravitational field of the galaxy over the molecular gas (or rather, what change it produces at the angular moment). This calculation has clearly shown that gas tends to move slowly toward the center, reaching annually the equivalent of the mass of the Sun to the nucleus of the galaxy. Therefore, the giant black hole of the galaxy M51 is in continuous ingestion of molecular gas and this transfer of matter has important consequences, as explained in the next section.
The quasars and core of M51 are related
Quasars are high energy density astronomical sources that emit huge electromagnetic energy from a small field. At first the origin of this energy was not clear. Today we know that its origin is the gas that falls to the huge black holes in the nuclei of galaxies. Around the black holes are created accretion discs, whose magnetic fields acquire great intensity, accelerating electrons and other elementary particles; they also create giant plasma jets observable by radio waves (Figure 3).
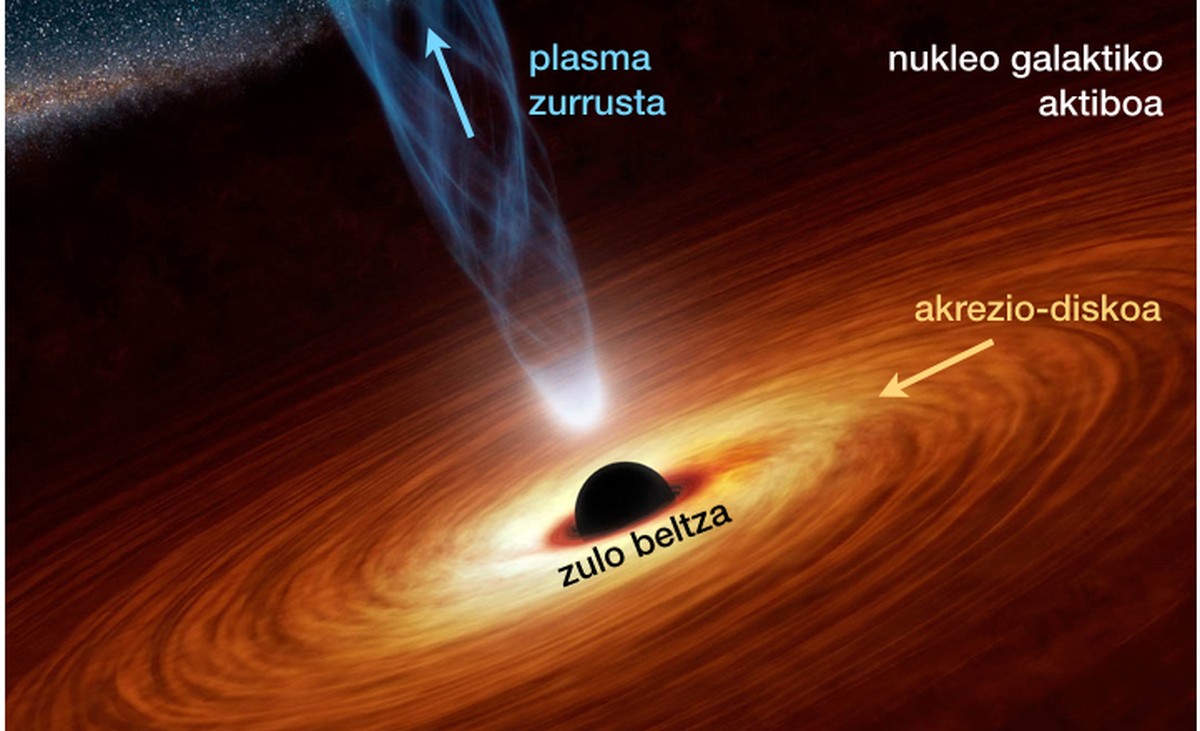
We know that in the nucleus of the galaxy M51 has developed an acrecion disk, since by radio waves we can see the plasma jet. Paradoxically, the most significant effect of this active nucleus is the release of molecular gas outside the nucleus: the transfer of mass reaching the black hole produces this opposite effect, aided by magnetic fields. Since NOEMA maps provide us with speed information, we can analyze this movement in detail and measure the mass being expelled. We could say that a certain dynamic equilibrium has been achieved in the nucleus: the fall of a small amount of gas to the black hole is the cause of most of the gas coming out of the nucleus; in extreme cases, the gas leaves the galaxy, which will totally limit the future of the galaxy. The main result of the thesis was the measurement of this rate of gas elimination, comparing with dynamic models the position and speed of the gas measured by the NOEMA interferometer, and concluding that this gas is moving outside the core at a speed of 300,000 km/h.
For the first time we have demonstrated in this thesis that the plasma jet, in addition to expelling gas from the environment of the huge black hole, alters the state of the gas. This jet consists of particles that move at a speed similar to that of light and, due to magnetohydrodynamic effects, increases the turbulence of the gas that passes through. At the same time, turbulence can prevent the collapse of gas: that is why there is no young star near the nucleus of the M51 galaxy, because the nucleus itself has transformed the gas into sterile. Figure 4 shows the location of the plasma jet and summarizes the effects it produces on the gas.
Even quasar can control the birth rate of stars thanks to such a mechanism. The active nuclei of galaxies, in addition to explaining the brightness of quasars, can condition the evolution of galaxies. If not, the galaxies would transform the gas much faster to form stars, and all the galaxies in our surroundings would have a red color (color of old and cold stars), without spiral arms, since it is impossible to keep the spiral arms without gas. Therefore, the interaction between giant black holes and galaxies is essential to explain the diversity of current galaxies, and observations made in the M51 galaxy have revealed the important details of this mechanism.
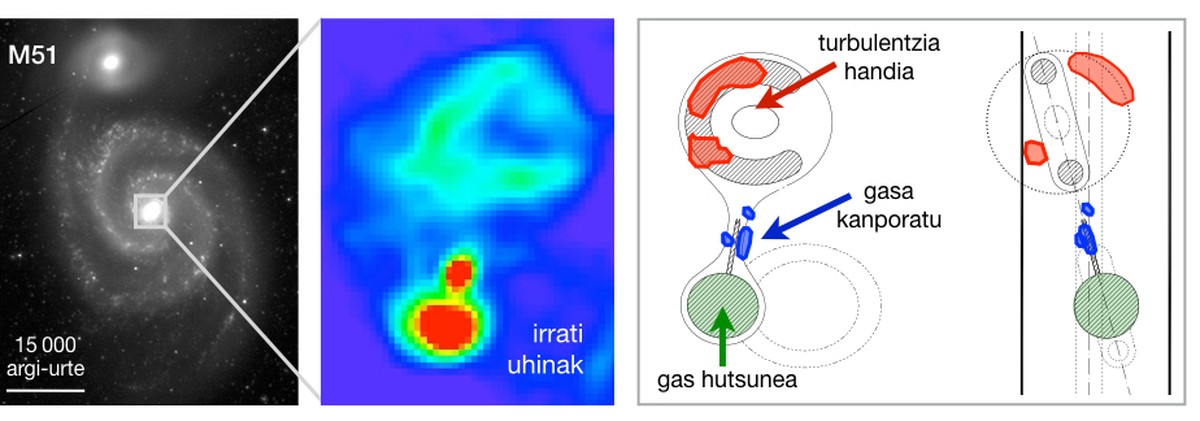
See and hear black holes
On 14 September 2015, the LIGO observatory detected gravitational waves generated by the union of two black holes. It was the first direct detection of gravitational waves, which were awarded the Nobel Prize in Physics Rainer Weiss, Barry C. Barish and Kip S. To Thorne scientists, for making possible observation of gravitational waves. This first detection was called GW150914, it is not a very poetic name, in reality, but the use of the date of the event facilitates the classification. These black holes collided had about 30 solar masses, which is a much smaller mass than that of the giant black hole we have studied in this doctoral thesis. On August 17, 2017 LIGO detected other gravitational waves, being the fifth detection but with a special feature of this GW170817. For the first time, in addition to receiving the signal from gravitational waves, scientists were able to detect this phenomenon across the entire electromagnetic spectrum. This encounter between two neutron stars revealed the existence of a new way of studying astrophysics: in addition to looking at the universe, gravitational waves that extend throughout space can be heard simultaneously.
As we have explained, the huge black holes have a great importance for galaxies, since controlling the position and state of gas condition the birth of new stars (and therefore, delimit the future of the entire galaxies). We can see black holes transversely and measure the influence of these monsters on galaxies. We still have to learn a lot about the behavior of this powerful motor that galaxies hide inside, but gravitational waves can help us. It would be fascinating to see and hear these mysterious nuclei of galaxies at the same time and complete the next beat of the symphony of black holes.
Bibliography
Sparke, L. S.; Gallagher, J. S. Galaxies in the Universe (Cambridge University Press, 2000).
Crane, P. C.; van der Hulst, J. M. (1992). “The radio jet in M51”, The Astronomical Journal, 103: 1146-50.
Fabian, A. C. (2012). “Observational Evidence of Active Galactic Nuclei Feedback”, Annual Review of Astronomy and Astrophysics, 50: 455-89.
Querejeta, M.; Meidt, S. R.; Schinnerer, E. et al. (2015). “The Spitzer Survey of Stellar Structure in Galaxies (S4G)”, The Astrophysical Journal Supplement, 219: 5, 1-19.
Querejeta, M.; Schinnerer, E.; Meidt, S. E. et al. (2016). “Gravitational torques imply molecular gas inflow towards the nucleus of M51”, Astronomy & Astrophysics, 588: 33, 1-19.
Querejeta, M.; Schinnerer, E.; García-Burillo, S. et al. (2016b). “AGN feedback in the nucleus of M51”, Astronomy & Astrophysics, 593: 118, 1-21.
Schinnerer, E.; Meidt, S. R.; Pety, J. et al. (2013). “The PdBI Arcsecond Whirlpool Survey (PAWS)”, The Astrophysical Journal, 779: 42, 1-29.
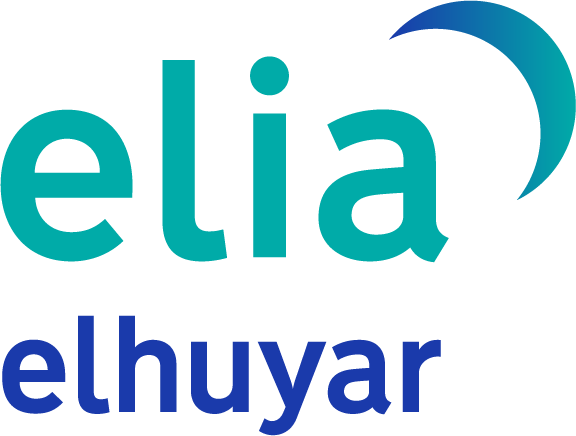
Gai honi buruzko eduki gehiago
Elhuyarrek garatutako teknologia