Nanomedicine as a treatment for breast cancer
2019/12/01 Sarasola Maizkurrena, Aintzane - Farmazian graduatua | Salvador Martinez, Aiala - EHUko Farmazia Teknologiako irakaslea | Hernandez Martin, Rosa Maria - EHUko Farmazia Teknologiako katedraduna | Igartua Olaechea, Manoli - EHUko Farmazia Teknologiako irakasle titularra | Santos Vizcaino, Edorta - EHUko Farmazia Teknologiako irakaslea Iturria: Elhuyar aldizkaria
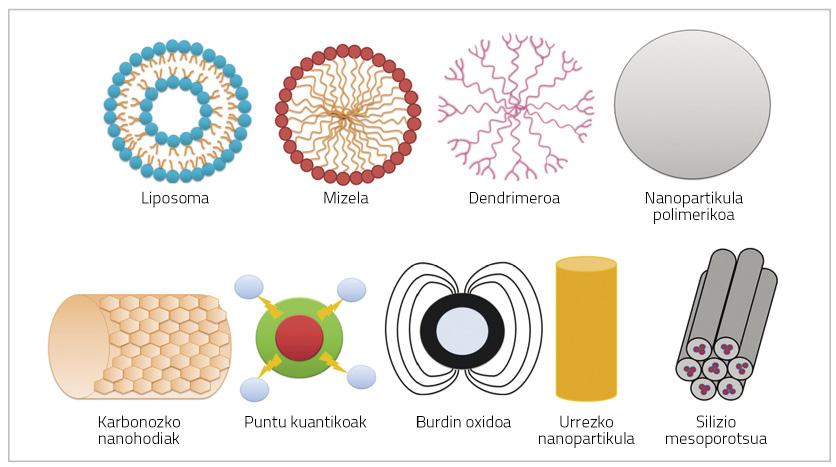
Breast cancer is the most deadly disease in women. The therapies currently used to fight cancer are mainly chemotherapy and radiation therapy [1, 2], and are highly invasive techniques that cause numerous undesirable effects [3]. Conventional chemotherapy affects the entire body, damaging both cancer and healthy cells. Therefore, the dose of chemotherapy is limited because it is a very toxic treatment. Nanoparticles can be appropriate therapeutic resources to overcome this lack of specificity of conventional chemotherapy [4].
Thus, using nanoparticles, it is possible to release the anticancer drug at the place of application or concentrate it on the target tissue reducing systemic toxicity. In addition, they solve the problems of some drugs such as low solubility or chemical instability. In addition, pharmacokinetics of drugs are improved and resistance of drugs used in chemotherapy is reduced [5]. As a result, nanotechnologies developed for the treatment of breast cancer have achieved greater efficacy and survival in breast cancer patients and reduced the unwanted effects of chemotherapy.
Characteristics and mechanisms of action of nanoparticles
Nanoparticles are very small particles (< 100 nm) that may consist of polymers, lipids, viruses or organometallic compounds (Figure 1) [6].
For the drugs used in the treatment of breast cancer to be fully effective it is necessary to take into account two factors. On the one hand, reaching the tissue in which the tumor is found as much as possible, and on the other hand, that the medicine that reaches the tissue directly affects the cancer cells. Both actions can occur through passive or active routing by nanoparticles (Figure 2) [4].
Passive routing
The drug is administered inactively and upon reaching tumor tissue is metabolized, activated and becomes a very toxic drug [7, 8, 9]. Passive routing can be performed in three ways:
a) Increased blood vessel permeability and retention effect (EPR)
As tumor cells and tumor tissues increase, their need for oxygen and nutrients increases. To meet these needs of oxygen and nutrients a new system of blood capillaries is created that does not develop completely and is therefore more permeable for certain particles of a certain size. That is why nanoparticles usually accumulate in tumor cells, which allows to increase the dose of medicine that will influence the inside of cancer cells without causing toxicity in healthy cells [4, 8].
b) Tumor microenvironment
This strategy considers the tumor environment. The drug is given to the conjugated patient (inactively) with a specific molecule of the tumor and, upon reaching it, the contour of the tumor makes it an active substance [10].
c) On-site administration
The direct application of the chemotherapeutic drug in the tumor prevents systemic toxicity and allows reducing the dose of the drug. For example, the administration of C mitomycin - conjugated dextran inside the tumor allowed to detect a higher concentration of the antichannel drug in the tumor area and a decrease in systemic toxicity [8].
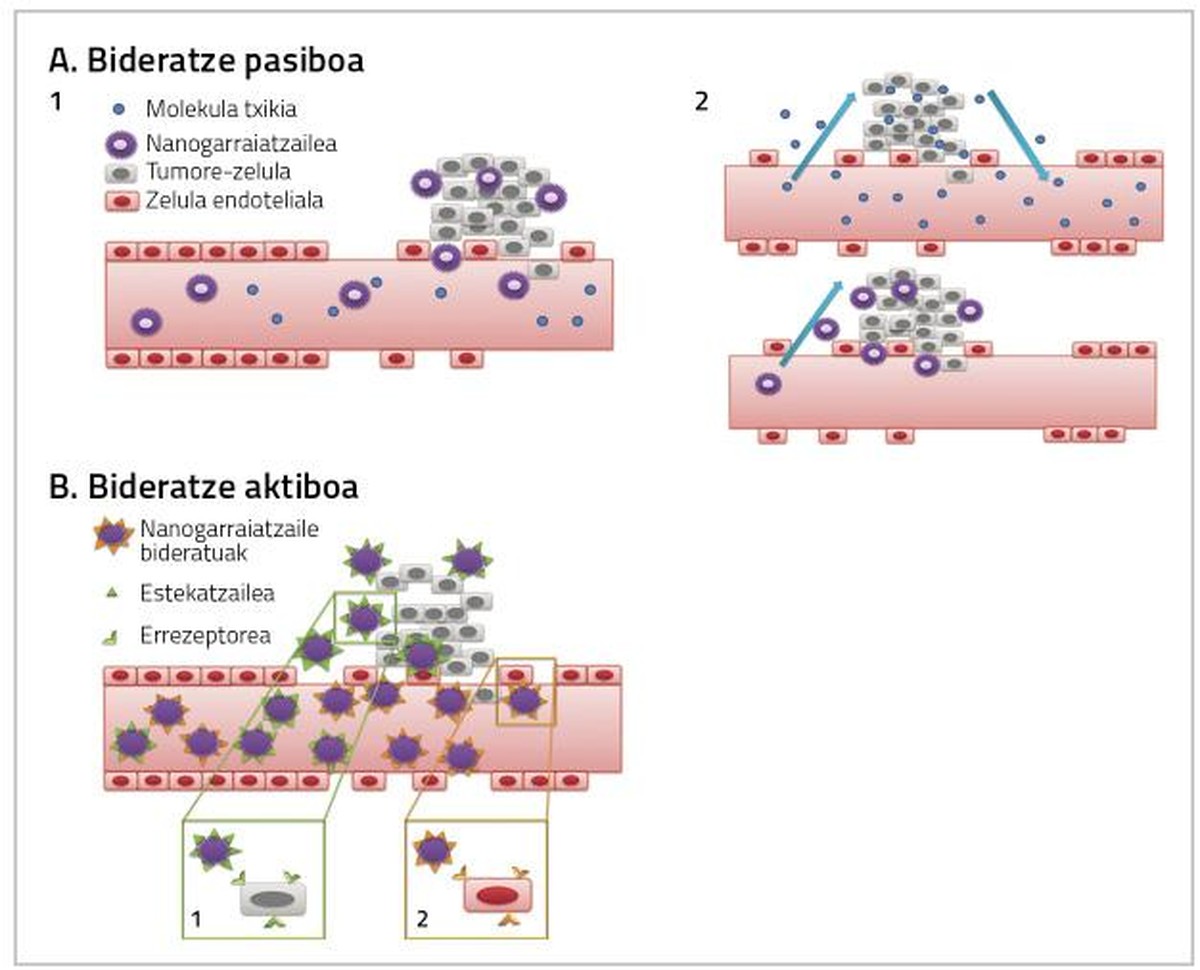
Active routing
The purpose of active routing is to interact the conjugated nanoparticles with the targets of the treatment area, so that the cancer tissue reaches a higher dose of drug (Figure 2).
Cancer cells have characteristics similar to healthy tissues. Therefore, for the separation of these cells specific binders are used to receptors that are overexpressed in cancer cells, generating specific interactions. Examples include the association of lectin carbohydrates, binder receptors, and antigen antibodies [8, 9, 11].
a) Carbohydrate orientation
One of the most important examples is that of lectine carbohydrate. Carbohydrates on the surface of tumor cells are not like normal cells. Lectin is able to recognize and associate glycoproteins present on the cell surface. Consequently, some carbohydrates interact with lectin, creating specific cell bonds. Lectins, therefore, can be molecules to transfer the drug to the cells or tissues it targets. Through this association of lectin carbohydrates two different nanoparticles can be developed. On the one hand, lectins can be integrated into nanoparticles and interact with carbohydrates present on the surface of target cells. This is known as direct lectin conduction. On the other hand, carbohydrates are integrated into nanoparticles to interact with the lectin present in target cells. This is what is called reverse scaling of lectins [4].
b) Conduction of receivers
The drug is conjugated with a polymer transporter that binds to the cells thanks to a binder that carries in the structure [12]. An example is the folate receptor. When a conjugate intended for folates on the cell surface binds to folate receptors, the plasma membrane collects this conjugate from the receptor and binder forming an endosome. Endosomal pH is acidified and as lysozymes are activated, the drug is released from the conjugate and introduced into the cytoplasm. Meanwhile, the released folate receptor returns to the plasma membrane to restart the process [4].
d) Antibody processing
This strategy is based on the action of monoclonal antibodies to orient nanoparticles towards tumor tissue [12]. Examples of this are immunoliposomes. Liposomes conjugated with antibodies form a specific link with the surface antigen of target cells, whereby the anticancer drug reaches tumor tissue (Figure 2) [13].
Final reflections
Given these possibilities, the efficacy of these nanomedicine can be predicted. However, there are few nanomedicine put on the market and still many existing for marketing are in clinical trials or pre-clinical phases. Although this means a lot of time and funding, the benefits that nanoparticles can report make it necessary to take into account their importance. Therefore, more research and development of new nanoparticles will be necessary, since in addition to making great advances in pharmaceutical technology and health, they can report a huge benefit to society.
Bibliography
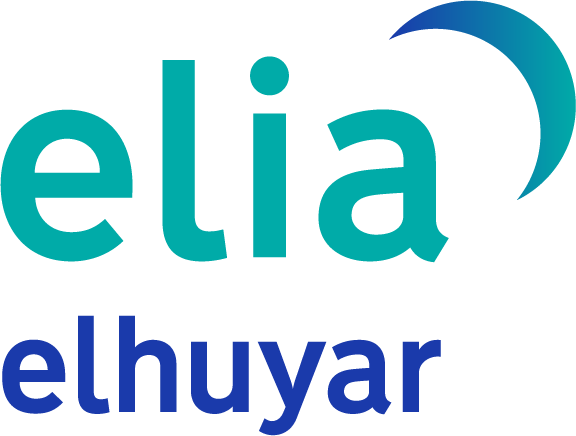
Gai honi buruzko eduki gehiago
Elhuyarrek garatutako teknologia