Black hole evaporation (Hawking effect)
1996/04/01 Campillo Robles, Jose Miguel - Fisika SailaZientzia eta Teknologia Fakultatea (EHU) Iturria: Elhuyar aldizkaria
Giving a classic view of black holes is not very difficult. The black hole is a body that swallows each and every one of the things that surround it through its violent gravitational field, even the fastest, like light. Nothing comes out of it, no radiation or anything else. That is, it absorbs everything and does not emit. In this way, it ingests non-stop energy and matter, increasing uninterruptedly. Therefore, the idea of sustainability arises from the impossibility of the black hole disappearing.
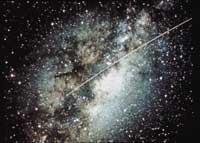
As for invisibility, due to the violent gravitational zone, the black hole cannot emit radiation, so it is invisible from the outside. Before moving forward, however, it should be nuanced. The matter that attracts the black hole moves at a vertiginous speed. The friction and impacts that occur in this process of fall are very intense and in this situation the atoms are not able to maintain their structure, becoming plasma. The plasma temperature is very high and emits mainly x-rays. As a consequence of this phenomenon, the environment of the black hole can be observed at a distance, but it is clear that this radiation does not correspond to the black hole but to the surrounding matter.
In 1974 this classic view of the black hole breaks, Stephen W. For the discovery of Hawking. During this time he was dedicated to investigating the quantum phenomena that occur around microscopic black holes, and proposed the existence of these bodies that were believed in the early times of the Universe. The result of research in this regard was that the black hole spontaneously emits thermal radiation.
As the gravitational field around black holes is so violent, how can it be emitted? To answer this question, we must use the principle that is one of the pillars of Quantum Mechanics, the uncertainty principle of Heisenberg. This principle was first postulated in 1927 and applies mainly to the microscopic world. One of his formulations tells us: “For relatively small times there is a very high uncertainty in energy” and mathematically expressed as:
... t * H
where H= h/2> (h, Planck constant), power deviation and t is the time interval. In other words, for any time it is not possible to know energy accurately and therefore, in the microscopic world, energy cannot take concrete values, such as zero. On the other hand, thanks to Einstein's theory of Special Relativity, we know that energy and matter are two sides of the same coin. Therefore, any energy fluctuation can become matter. That is, from the principles of Quantum Mechanics it follows that in a very short time matter can emerge from nothing. Therefore, the vacuum of Quantum Mechanics is not the state of the lack of field, particles and energy, as occurs in Classical Mechanics, but the state of lower energy. Therefore, it can be said that the void is not empty.
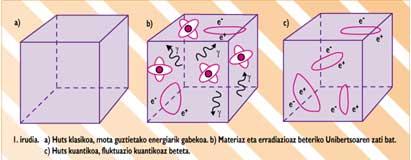
In quantum vacuum, pairs of antiparticle particles are constantly forming. These couples are called vacuum fluctuations and their half-life is very short. For example, the distance between the formation and destruction of the pair of electrons and positrons is between 10 and 21 seconds and two thousand times less than the life of the pair of protons and antiprotons. Why are there differences of this kind? The proton is heavier than the electron, so the energy of fluctuation must be higher to produce proton, so the average life of heavy particles will be lower.
Since these times are very low, fluctuations cannot be detected directly. Therefore, the particles of fluctuations are called virtual. However, the presence of these particles can be demonstrated through phenomena derived from their own influence, such as the Lamb sliding effect. In this effect, the energy levels of the hydrogen atom suffer a slip due to the influence of virtual pairs.
After that, the prudent reader may think what relationship exists between radiation from any black hole and quantum fluctuations? To try to clarify it, as Hawking did, we must analyze the fluctuations around the black hole itself. When created around the black hole, virtual couples suffer a strong gravitational force, but this process is not the same in all cases.
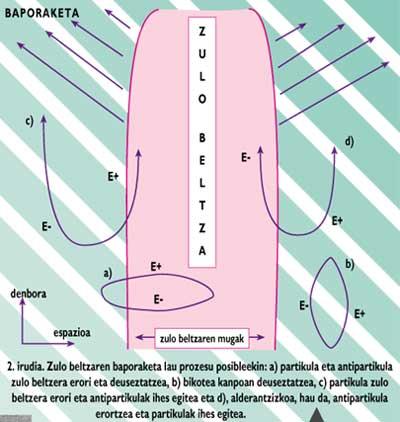
The gravitational force varies a lot at a very short distance around the black hole, so the influence on each of the components of this pair is different. The increase of this difference will allow to divide the parts of the couple, which will mean a break of the structure of the couple. In these cases, virtual particles become real and therefore measurable. This vacuum situation is called vacuum polarization.
When the particle particle couple structure is formed next to the black hole, four processes can occur (see figure 2): a) falling and removing particles and antiparticles to the black hole, b) external removal of the pair, c) falling of the particle to the black hole and d), upside down, that is, the fall of the antiparticle and the leakage of particles.
Hawking studied these four options and calculated the odds of each of them. According to their evidence, the most likely process is the last. This indicates that the number of antiparticles falling into the black hole is greater than that of particles. If we come to analyze its consequences. On the one hand, as antiparts have negative energy, the energy of the black hole is decreasing and with it its mass. If we look at this phenomenon from the outside, we can think that those escaping particles are expelled by the black hole. If analyzed externally, the black hole is observed to “evaporate” and emit particles.
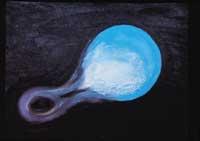
Here is Hawking's discovery: the vaporization of black holes. But it was more Hawking and he postulated that the body has a temperature. The temperature corrects the emission mode, that is, the emission spectrum is thermal and is equal to that emitted by the “dark object” with the same temperature. In addition, the ratio between the temperature of the black hole and the mass is inversely proportional (see graph 1). Thus, the lower the mass, the higher the body temperature and, if the mass is large, the temperature will be low.
For example, a microscopic black hole of one billion tons will have a temperature of 10 12 K. By increasing the temperature, considering that the emission will be higher, the small mass black hole will radiate much more than the large mass, thereby losing your mass much faster. By losing the mass, the temperature increases and thereby the radiation increases. The acceleration of radiation is a consequence of all this. And finally, what will happen when the mass of the black hole per emission is very small? To answer this we would need the Quantum Theory of Gravitation, and not having it, it is not easy to answer. Some theories argue that the black hole will disappear by a large explosion of radiation, which would apparently have the power of millions of H bombs.
On the other hand, the temperature of the black holes of greater mass is negligible. The black hole of a solar mass will have a temperature of 10 -7 K, much lower than that of the background radiation that forms the Universe (2.7 K), so the black hole will be smaller than that of absorption. Therefore, until the temperature around it is lower than that of the black hole, the black hole will gain mass and energy, the quantum effect being negligible.
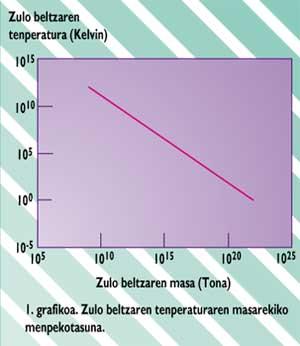
Vaporization is therefore a quantum effect, so it is important for microscopic black holes. But it is applied in all black holes, both rotation and electric charge. Black hole research can be more important if we consider, as some researchers believe, that it could serve to obtain the Quantum Theory of Gravitation.
In the explanation of this effect three large areas of Physics come together: Gravitation, Thermodynamics and Quantum Mechanics. This tells us that the sum of Physics is profound, although it is often not so. Since the degree of specialization of scientists is increasing, these principles are practically forgotten today. Do we ever recover them?
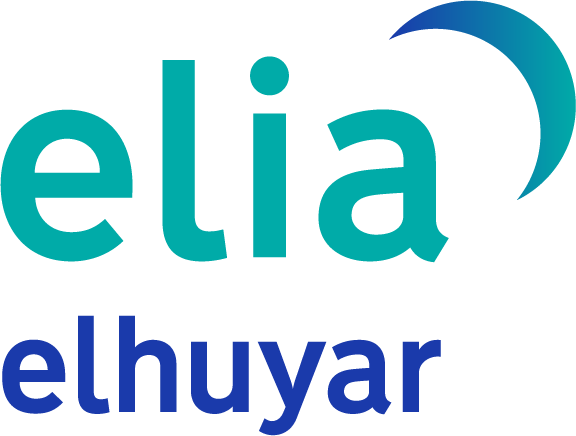
Gai honi buruzko eduki gehiago
Elhuyarrek garatutako teknologia