Neutrinos: revealing the secrets of antimatry
2021/12/01 Esteban Muñoz, Ivan - Fisikako ikertzailea Iturria: Elhuyar aldizkaria
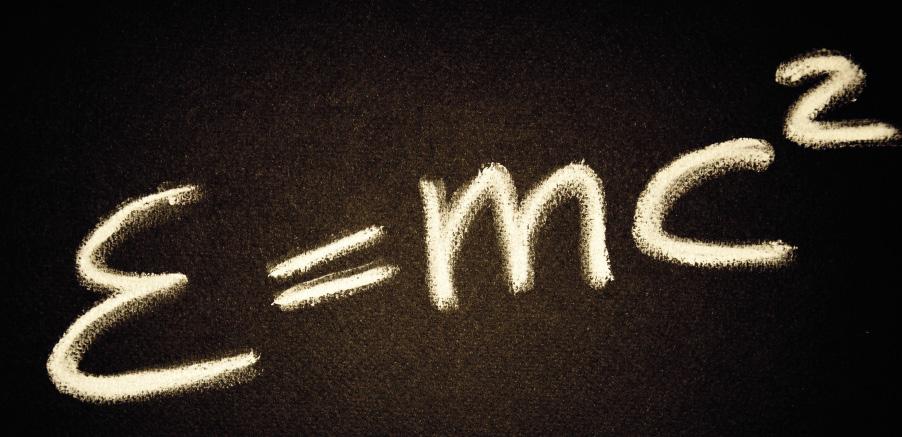
When and how does our universe arise? Why is it full of matter? These are questions that humanity has always pondered. And, surprisingly enough, we live at a time when science has begun to answer those questions.
For this, in the last century courageous theoretical ideas, gigantic experiments and luck have been necessary. In recent years, scientists have been investigating a fleeting particle, neutrin, to find answers to these questions.
We are the dust of a Great Explosion
According to the data we have, our universe began in a big bang, called the Big Bang, with incredible energy: a trillion energy for every cubic centimeter of more than one atomic bomb. All this energy generated the current matter, as Einstein's famous formula explains: E = mc2. The formula allows to transform energy into mass. Or vice versa, with a huge amount of energy, create mass.
However, this theory has a big problem. XX. At the beginning of the 20th century, scientists discovered some curious particles that looked like common matter, but when faced with common matter, they generated a glow of light that destroyed each other. This was called an antimatry and as it was discovered that all the particles had an antiparticle. Moreover, in all experiments, materials and antimateries seemed inseparable: if all the particles in a system were exchanged with their antiparticles, the behavior of the system did not vary at all.
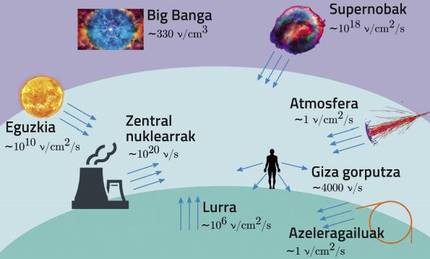
So if particles and antiparticles are inseparable, the Big Bang would produce the same number of particles and antiparticles. Then they would be destroyed and then they left the universe full of light. And there would be no matter left to form galaxies, stars, planets and ourselves.
Looking for differences between matter and antimatry
For this paradox, XX. From the second half of the 20th century, most scientists believed that matter and antimatry are not exactly the same. The slightest difference would also generate more particles than antiparticle at Big Bang, all antiparticles would be destroyed with particles and the remaining particles would form the current universe. But only experiments take science. Fortunately, in 1964, an American research group discovered that some of the particles generated in nuclear reactions and their antiparts disintegrate differently. However, as they were further researched, all the experiments had the same conclusion: the difference found was too weak to explain the large number of particles in our universe.
So in an attempt to understand our universe, science focused on other particles: neutrines. Neutrinos are the lighter and fleeting particles found by science. Despite its abundance in nuclear reactions, it took 25 years from its theoretical proposal to its experimental discovery due to the weakness of its interactions. And that is, although a hundred trillion neutral from the Sun every second pass through our bodies, in our lifetime only one of them will collide with us on average. So large detectors are needed to investigate neutrinos: the more material in the detector, the more likely a neutrino is to interact with the matter. Current detectors are larger than intercontinental planes! Although fleeting, neutrinos are easy to create. Look at the Sun and you will receive trillions neutrinos. Go to a nuclear power station and get a million times more neutrino to your detector. A beam of protons collides with a dense material creating a ray of neutrino.
Like all particles, neutrinos have an antiparticle: the antineuthrin. And in recent years, the differences between neutrinos and antineutrins have been appearing in experiments.
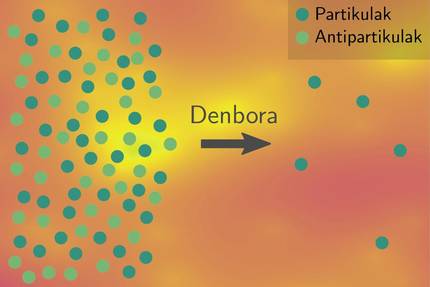
Neutrinos: the history of the three flavors
To understand these differences, you have to understand an important property of neutrinos: taste. According to particle theories and experiments, there are three types or flavors of neutrino. Each flavor forms in a different reaction, with different interactions. Moreover, theoretically a neutrino never alters its taste.
Or that's what scientists believed. XX. At the end of the 20th century, several giant experiments were done to detect neutrinos from the Sun and cosmic rays. The taste of these neutrinos is very well understood theoretically, but to the detectors some neutrinos came with another taste. What's more, the more distances they traveled, the more neutrinos their taste changed. This fact surprised scientists and forced them to change theoretical models.
To explain the results of these experiments, it was proposed that neutrinos had a small mass. It was the simplest explanation and a small mass (10-37 kg approx. A million times less than an electron) was enough to understand the taste changes of neutrinos. When scientists began to investigate this proposal, they realized a surprising conclusion: if the mass of neutrinos caused taste changes, it would be possible for neutrinos and antineutrins to change flavor differently. That is, neutrinos offered a new opportunity to investigate differences between matter and antimatry.
Understanding antimatry with neutrino experiments
As more was known about the taste changes of neutrinos, it was increasingly evident that neutrinos and antineutrins could change the taste differently. And that could explain why our universe is full of matter. To better understand this, data is currently being taken from two experiments: NOnA in the US and T2K in Japan. In these experiments, a beam of protons collides with a piece of graphite forming particles. The disintegration of these particles generates a high number of neutrinos and antineutrins. Moreover, particles generated by protons when they collide with graphite can be controlled by magnetic fields so that only neutrinos or antineutrins are disintegrated.
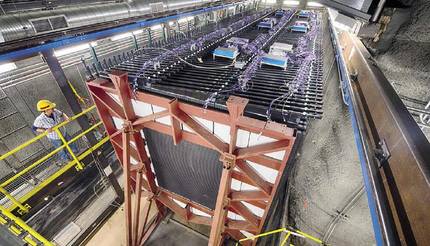
This allows the production of neutrino or antineutrine rays. They cross between 500 and 800 kilometers underground and are detected in giant detectors. Once the results of these experiments have been analysed, it is possible to know whether neutrinos and antineutrins alter the taste.
However, a single experiment is not sufficient to obtain the final results. For this, it is necessary to combine the data of many experiments in a single theoretical model. And that's the basis of my thesis. First, I've developed T2K and NOnA experimental simulations to understand their results within a common framework. After matching the results of other neutral experiments in recent decades, everything we know about the taste changes of neutrinos can be quantified with statistical instruments.
The result of this analysis was the difference between neutrinos and antineutrins. Moreover, this difference is quite large: A thousand times stronger than discovered in 1964! So this result is surprising and exciting: neutrino may be the key to the difference between matter and antimatry at the Big Bang.
However, understanding the results of these experiments is not easy. Before they get to the detector, the neutrinos are hundreds of kilometers. According to our theories, this should not have much influence on the results. But neutrinos already nullified theories 30 years ago: it is essential to analyze the data without theoretical prejudices. And if neutrinos interact strangers with matter, the data may have to be interpreted differently.
That was the second part of my thesis. I've studied the interactions of neutrinos with matter and whether other experiments have nullified those interactions. Moreover, new interactions can cause differences between matter and antimatry. Understanding this is also fundamental to understanding the differences between neutrinos and antineutrins.
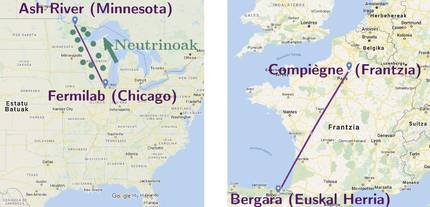
So I've been able to analyze all the data from the experiments in more general theoretical models. This has had an interesting result: it seems that what experiments have discovered at the moment is that neutrinos and antineutrins are different, not the consequence of other interactions. To conclude this, it has been important to have carried out several experiments with neutrinos so far and will be essential in the future.
It is interesting that in the Basque Country some basic elements are being made for such an experiment. In a few years, the European Neutron Spalting Source (ESS) will be built in Sweden, with the participation of companies in the Bilbao area. ESS will create a neutron ray to investigate material science. But the same neutron generation process will generate eleven neutrinos. These neutrinos will not alter their taste or serve to analyze the difference between matter and antimatry. But part of my thesis has shown that with the right detectors for those neutrinos, we can perfectly explore their interactions. This will allow a better understanding of the experiments on changes in the taste of neutrinos and thus the differences between neutrinos and antineutrins.
And that's not the only connection between neutrino technology and the Basque Country. Although the investigation of neutrinos appears to be far from the reality, specific detectors have been developed for the observation of these particles. The DIPC PETALO project aims to use these specific detectors in nuclear medicine. The vaccine is also knowledge of neutrinos!
So little by little, the human being knows more about the beginning of the universe. The mystery of antimatry is revealed by particle experiments. In recent years, it seems that neutrinos can be the key, and for that they're building several experiments around the world. What will we learn from them and what new questions will bring us the results... who knows!
References
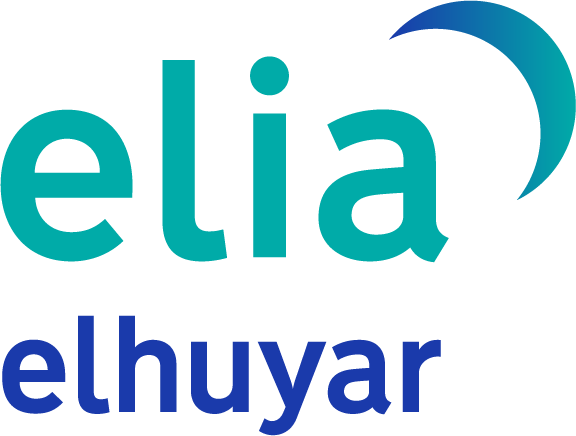
Gai honi buruzko eduki gehiago
Elhuyarrek garatutako teknologia