Interstellar dust
1987/10/01 Arregi Bengoa, Jesus Iturria: Elhuyar aldizkaria
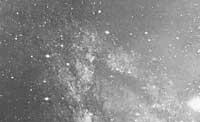
In this issue we will try to consolidate a point that has not been tied well in the previous one. When talking about the arguments of those who defend that the first steps of the prebiotic evolution that would imply the birth of life occurred in space, we did not take into account a theory to explain the formation of these compounds. Since this alternative process of formation of these basic organic compounds is essential to take into account the aforementioned theory, then we will try to fill this gap. At the same time, we will present this work as a first approach to another topic that we will develop later. It is the amount of dark matter in the Universe and its influence on the evolution of the Universe.
Until the beginning of this century, astronomers considered art to be nothing or the most absolute void. The first evidence of the existence of interstellar matter was not achieved until 1904, J. Thanks to the work of Hartmann. XVIII. The dark phangos, known in the sky since the end of the twentieth century, were considered true gaps and were not considered as a consequence of the absorption of the stars and the light proper to the dust that can exist between us until the third decade of this century.
Before moving forward we will define what we mean by interstellar matter. It is necessary to differentiate gas and dust from the first interstellar. Gas is gas, namely hydrogen and helium. Under conditions in interstellar space, these gases cannot be condensed (even if the temperature is only 3K). However, as already indicated, within the stars there are other solid fractions formed by other heavier elements that have occurred as a result of thermonuclear reactions: interstellar dust. The components, in particular, are oxygen, carbon, nitrogen, magnesium, silicon and iron (along with the hydrogen they can ingest), in a decreasing order of abundance.
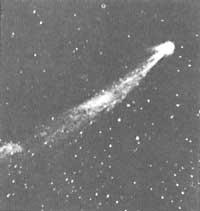
According to studies on the star within our Galaxy, the mean density of interstellar matter is approximately one hydrogen atom per cubic centimeters. But the distribution is not uniform, since matter accumulates in clouds. The most abundant element in clouds is, of course, hydrogen. They can appear mainly in two different forms: forming aIa molecules in the atomic state. The first case occurs when clouds are very low density: diffuse clouds. Thus, the ultraviolet emission of stars, although the process is not very effective, is sufficient for hydrogen not to form molecules. These clouds are identified by the 21 cm line they form in the spectrum. On the contrary, when the clouds are denser, ultraviolet rays are usually absorbed on the outside, without passing inland.
Then hydrogen remains in molecular state. Contrary to what one might think, the formation of molecules H 2 is also ineffective. The clashes between the hydrogen atoms present in space are ineffective, that is, they do not develop enough energy to overcome the electric repulsion that occurs when the atoms approach. The bond is only formed when the shock occurs on the surface of a fraction of dust; (when one atom of H touches another on the surface of the fraction). The density of these molecular clouds can reach 10 6 atoms/cm 3. Therefore, much less than can be achieved in terrestrial laboratories. Even so, in no case can we underestimate the role of this matter. The interstellar space and the enormous measures we have to work on researching these clouds compensate for low density. Moreover, the dust supply that can have a typical interstellar cloud is about 2% of its mass.
We have said above that the existence of interstellar dust began to be discussed at the beginning of the century. Until the 4's, hypotheses were not made about its origin: In 1935 B. The Lindblad dust fraction supported condensed matter in space itself and argued that by the mid-century H.C. van de Hulst could be ice. This hypothesis presented a difficulty: the low value of interstellar pressure prevents condensation of the aforementioned elements, unless it is on other pre-existing fractions.
Until the 7's these basic fractions were not found until it was confirmed that they were surrounded by a fraction of several silicate stars. These silicate cores are formed on the surface or atmosphere of the stars and then go further into another cloud by the pressure of radiation, taking back the temperature and pressure in the open space. This is the seed of interstellar dust fractions and with it begins the life cycle of the fraction. On this seed the most abundant elements are condensed; the thighs (H 2 0, CH 4, NH 3) formed by the hydrogen mixture on the surface of the fractions (O, C, N).
The imprint of the next development step was given by spectroscopic studies of several clouds. Faced with what was expected, the water could be quite abundant in many clouds, especially in diffuse ones. In this case it is also due to ultraviolet emissions. These can break the newly formed molecules by giving radicals. These are, in principle, very reactive and can be joined to give quite complex compounds in the first steps of the evolution of the powder fractions. Figure 1 presents a scheme of this process along with the list of the most numerous molecules obtained.
At this point it is interesting to cut the dispersion of evolution to explain one of the experimental techniques that have been used to analyze the cycle.
Leiden J. Astrophysics Laboratory Mayo Greenberg and his colleagues managed to partially simulate the conditions of space inside a camera. They managed to lower the temperature to 10K by a liquid helium cryostat. They could lower the pressure from 10 to 8 bulls (in space can be 10 to 19 Tor and less). Through a camera window the ultraviolet radiation is introduced and from a tube different gas mixtures can be introduced, being the bases CH 4, CO, H 2 O, C0 2, NH 3, N 2 and 0 2. Of course, the time scale is huge: one hour of laboratory radiation equals a thousand hours of radiation from a cloud.
The experiments carried out on the attic simulating as best as possible the conditions of the diffuse clouds, in addition to confirming the steps described so far, have given rise to other conclusions. On the one hand, it is logical to think that this phase of low activity of diffuse clouds is the longest in the fraction cycle, but there are also many processes that can heat the dust (for example, the formation of a star around).
Therefore, the laboratory has also analyzed this possibility, with two types of conclusions. In the first case, when the cloud warms very slowly, the increase in activity between radicals is high, producing a residue that we will call yellow matter. Although its components are not yet known, it is supposed to consist of a complex organic matter, the most important feature being stability, as it remains up to 450 K without evaporating. In the second case the cloud heats up faster and then the reactions between radicals are much faster. These reactions further reinforce the energy process they release until explosions occur, where the temperature rises to 25K.
Again, we can follow the thread of life from the dust fractions. The warming that clouds can suffer is usually slow. Only when the star has to be produced in the cloud itself can the temperature increase so that the explosions mentioned are generated. This second case is a later phase of the cycle and we will see later what happens in this case. In general, therefore, it can be considered that the dust silicate core is surrounded by a layer of yellow matter (see Figure 2(a)). The condensation process can be much easier when the diffuse cloud, for any reason, reaches enough density to become a molecular cloud. Consequently, on yellow matter a new layer is formed, but now the conditions do not allow it to transform (see Figure 2(b))).
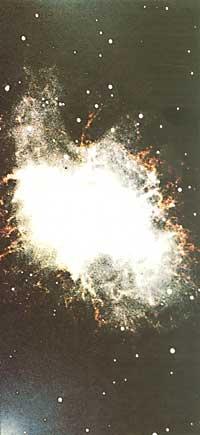
The last phase occurs when the cloud starts shrinking to give stars. On fractions that will not be part of the star, the ice forms a second outer layer, since the conditions are more suitable in less time than before. When the star is lit, the nearest parts will evaporate the outer layers and the others will suffer different erosion or erosion processes. One of them is the explosions mentioned above.
The radicals and temperature increase produced by the radiation of the newborn star contribute to the reaction of the chains and are the basis of the explosion. Consequently, the fraction loses the entire outer layer, leaving the yellow matter. Finally, the push of ultraviolet radiation expels all fractions to form the diffuse cloud again. It is then the conditions described at the beginning and fractions will only store yellow matter to start a new cycle.
These are therefore the main axes of the evolution of interstellar dust and the theory of the formation of complex organic compounds that subsequently fell on Earth (e.g. through comets).
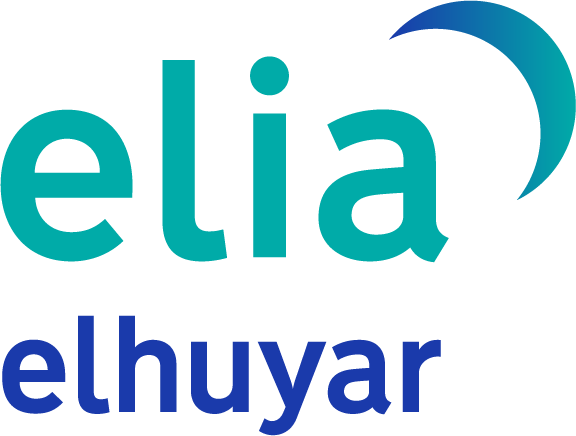
Gai honi buruzko eduki gehiago
Elhuyarrek garatutako teknologia